RESEARCH
Our research vision
Carbon is the backbone of Life on Earth, due to its ability to form complex molecules such as DNA or proteins. Most of Earth’s carbon is stored in rocks and sediments, the rest is in the ocean, atmosphere, and in living organisms, constantly being cycled between each reservoir via the biogeochemical process called carbon cycle. The unprecedented levels of energy consumption, mainly via fossil fuel combustion, are disrupting the carbon cycle and are leading to accumulation of CO2 in the atmosphere. This poses a severe threat to our planet, destabilizing climate.
Photosynthetic microalgae are crucial to the carbon cycle by fixing CO2 into organic carbon and this is why they are key players of the planetary metabolism drawing down carbon. Our vision is that by mastering the regulatory mechanisms controlling photosynthetic CO2 metabolism we can empower the biosphere to respond more effectively to regulate atmospheric CO 2 concentration and we advance towards a sustainable low carbon economy. For this we are investigating how CO2 acts as substrate for photosynthesis and as signalling molecule in conjunction with light to control cellular homeostasis in microalgae.
Introduction and state of the art
Light carries fundamental information perceived by photoreceptor proteins, whose role is to convert light, a physical signal, into biological signals that regulate physiological and developmental processes [1]. Light also provides the energy that fuels photosynthesis to fix inorganic carbon into organic carbon that feeds nearly all life on Earth. Nevertheless, excess light can be toxic and lead to the formation of harmful reactive oxygen species that result in cell death. This is prevented by the photoprotection mechanism qE (energy quenching) that converts the excess absorbed light energy to harmless heat instead of channelling this energy towards photosynthesis. In the green model microalga Chlamydomonas reinhardtii (hereafter Chlamydomonas) the protein Light Harvesting Complex Stress Related 3 (LHCSR3) is the major molecular effector of qE [2] while LHCSR1 can also contribute significantly in qE under conditions where LHCSR3 is not expressed [3].
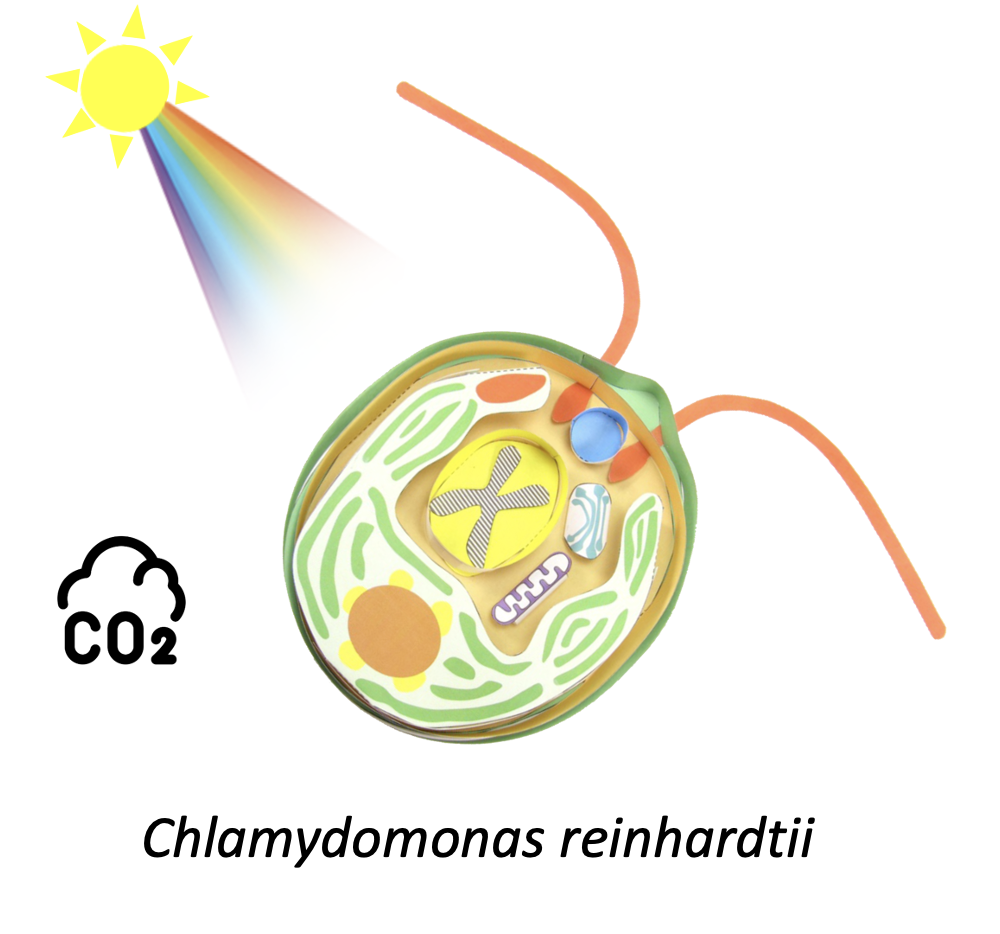
Algae also need to deal with limiting CO2 availability that often occurs at their habitats, despite the abundance of CO2 in the atmosphere, e.g. due the low solubility of CO2 in aquatic environments. Under low CO2 conditions, most algae activate a cellular CO2 concentrating mechanism (CCM) to elevate the level of CO2 at the site of fixation by Ribulose-1,5-bisphosphate carboxylase/oxygenase, and avoid growth limitation. In Chlamydomonas, CCM mainly comprises of carbonic anhydrases (CAHs) which interconvert all forms of inorganic carbon (Ci), and high affinity transporters that bring Ci into the cell. Almost all CCM-related genes are under the control of the nuclear factor CIA5 [4,5].
Integrated view of light and metabolic signalling in algae
Interdisciplinarity is the key to expanding frontiers and breaking barriers in science, prerequisites of advancing our understanding of how life is organized and regulated. Our research relies on this principle and aims to reach an integrated view of light and metabolic signalling in algae. Photosynthetic processes are usually ignored when focusing on photoreceptor research, and vice versa; same is true for photoreceptor vs metabolism research or CCM vs qE-related questions.
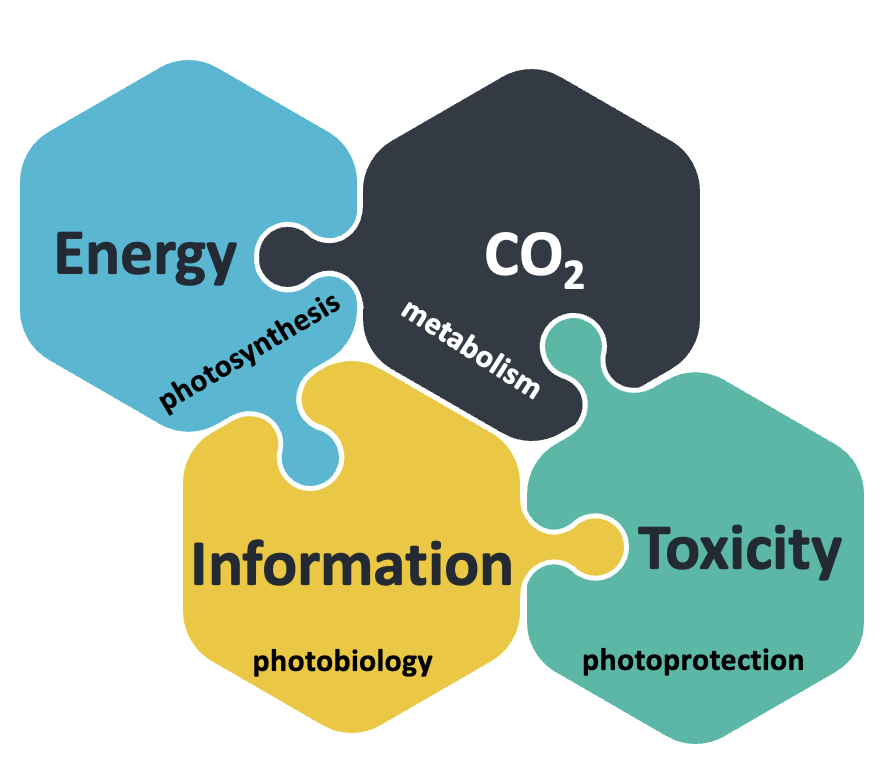
Our work bridges the gap between those research fields. Indeed, we build on our recent work that discovered i. direct molecular links between cytosolic photoreceptor (PHOT) signalling, qE and starch metabolism in the chloroplast, ii. a tight transcriptional interconnection of qE and CCM and iii. an intriguing new role of CO2 as signalling molecule activating light-responsive gene expression in the dark. We are aiming at creating a broad and in-depth molecular understanding of the fundamental biological processes of photoperception, photoprotection and carbon metabolism (CCM or starch accumulation).
We are combining genetic, molecular, biochemical, physiological and mathematical modelling approaches to identify factors (metabolic, transcriptional, post-transcriptional) involved in sensing and responding of Chlamydomonas to changing light and CO2 availability and in regulating metabolism and carbon partitioning. We are aiming at to ultimately reaching a systems view of photosynthetic dynamics in microalgae, that would allow us to fine-tune (at the molecular level) light energy management, carbon partitioning and biomass yield and composition.
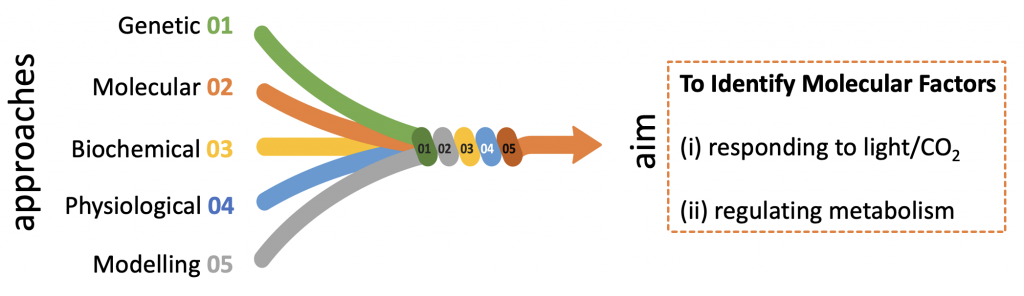
Recent highlights from our research activities
References
- Jiao, Y., Lau, O. S. & Deng, X. W. Light-regulated transcriptional networks in higher plants. Nat. Rev. Genet. 8, 217–230 (2007).
- Li, Z., Wakao, S., Fischer, B. B. & Niyogi, K. K. Sensing and responding to excess light. Annu. Rev. Plant Biol. 60, 239–260 (2009).
- Dinc, E. et al. LHCSR1 induces a fast and reversible pH-dependent fluorescence quenching in LHCII in Chlamydomonas reinhardtii cells. Proc. Natl. Acad. Sci. U.S.A. 113, 7673–7678 (2016).
- Moroney, J. V. et al. Isolation and Characterization of a Mutant of Chlamydomonas reinhardtii Deficient in the CO2 Concentrating Mechanism. Plant Physiol. 89, 897–903 (1989).
- Fukuzawa, H. et al. Ccm1, a regulatory gene controlling the induction of a carbon- concentrating mechanism in Chlamydomonas reinhardtii by sensing CO2 availability. Proc. Natl. Acad. Sci. U.S.A. 98, 5347–5352 (2001).
- Petroutsos, D. et al. A blue-light photoreceptor mediates the feedback regulation of photosynthesis. Nature 537, 563–566 (2016).
- Redekop P, Sanz-Luque E, Yuan E, Villain G, Petroutsos D, Grossman AR. Transcriptional regulation of photoprotection in the dark-to-light transition – more than just a high-light matter. Science Advances 8, eabn1832 (2022).
- Ruiz-Sola, M. et al. Photoprotection is regulated by light-independent CO2 availability. Nature Communications (2023, accepted). https://doi.org/10.1101/2021.10.23.465040.
- Arend M, Yuan Y, Omranian, N, Nikoloski Z, Petroutsos, D. Widening the Landscape of Transcriptional Regulation of Algal Photoprotection. Nature Communications (2023, accepted) https://doi.org/10.1101/2022.02.25.482034.
- Yoshioka, S. et al. The novel Myb transcription factor LCR1 regulates the CO2-responsive gene Cah1, encoding a periplasmic carbonic anhydrase in Chlamydomonas reinhardtii. Plant Cell 16, 1466–1477 (2004).
- Klein, U. Intracellular Carbon Partitioning in Chlamydomonas reinhardtii. Plant Physiol. 85, 892–897 (1987).
- Yizhong Yuan, PhD thesis. Phototropin links blue-light perception to starch accumulation in Chlamydomonas (2022) https://tel.archives-ouvertes.fr/tel-03917166.